Radiation Hazards: Difference between revisions
Jess Brewer (talk | contribs) |
mNo edit summary |
||
(22 intermediate revisions by 3 users not shown) | |||
Line 1: | Line 1: | ||
{{subpages}} | {{subpages}} | ||
{{Image|XKCD Radiation Chart.png|right|350px|Fig. 1 Handy chart from [http://xkcd.com/radiation xkcd.com] compares radiation doses.}} | {{Image|XKCD Radiation Chart.png|right|350px|Fig. 1 Handy chart from [http://xkcd.com/radiation xkcd.com] compares radiation doses.}} | ||
{{Image|Electrify5Radiophobia.009.jpeg|right|350px|Fig. 2 Effects of free radicals on DNA.}} | {{Image|Electrify5Radiophobia.009.jpeg|right|350px|Fig. 2 Effects of free radicals on DNA.}} | ||
'''Radiation hazards''' are not as easy to understand as anyone would like. A primary obstacle to gaining public acceptance for [[Nuclear_power_reconsidered|reconsidering improved nuclear power]] is a generalized [[Fear of radiation|fear of ''radiation'']]. People have difficulty distinguishing between a ''little'' and a '''lot''', so that this has often been oversimplified to, "The only acceptable amount of radiation is ''zero''!", a goal that cannot be met because we are constantly subjected to natural radiation over which we have little control, and because all living beings are themselves inherently somewhat radioactive. A sensible understanding of radiation dangers needs to be based on the type of radiation, its intensity, and the time over which the exposure is delivered. This article is an attempt to describe what sorts of damage which kinds of radiation can do, how much exposure over how long a time is required to damage human health, and how quickly the damage caused by radiation can heal.<ref>[https://jick.net/skept/RadHaz/RadHaz_talk.pdf Lecture on Radiation Hazards] Jess H. Brewer</ref> | |||
Figure 1 compares a range of radiation exposures, from the dose received from sleeping next to another human for a night (0.05 <math>\mu</math>Sv) to the usually fatal dose of 4 Sv, a hundred million times greater. '''''Warning''''': such comparisons can be misleading unless one understands that some are "delivered all at once" and others are "accumulative" over times up to a year. There is great controversy over the latter, since DNA usually "heals" strand-breaks due to free radicals produced by ionizing radiation (see Fig. 2) within hours or days,<ref>[https://www.ncbi.nlm.nih.gov/pmc/articles/PMC4060780/ "Effects of Ionizing Radiation on Biological Molecules—Mechanisms of Damage and Emerging Methods of Detection", Julie A. Reisz, Nidhi Bansal, Jiang Qian, Weiling Zhao, and Cristina M. Furdui] doi: 10.1089/ars.2013.5489</ref> suggesting that whole-body doses may '''not''' "accumulate" over longer periods. | Figure 1 compares a range of radiation exposures, from the dose received from sleeping next to another human for a night (0.05 <math>\mu</math>Sv) to the usually fatal dose of 4 Sv, a hundred million times greater. '''''Warning''''': such comparisons can be misleading unless one understands that some are "delivered all at once" and others are "accumulative" over times up to a year. There is great controversy over the latter, since DNA usually "heals" strand-breaks due to free radicals produced by ionizing radiation (see Fig. 2) within hours or days,<ref>[https://www.ncbi.nlm.nih.gov/pmc/articles/PMC4060780/ "Effects of Ionizing Radiation on Biological Molecules—Mechanisms of Damage and Emerging Methods of Detection", Julie A. Reisz, Nidhi Bansal, Jiang Qian, Weiling Zhao, and Cristina M. Furdui] doi: 10.1089/ars.2013.5489</ref> suggesting that whole-body doses may '''not''' "accumulate" over longer periods. | ||
Line 32: | Line 31: | ||
- AM Radio, FM Radio, Shortwave Radio, TV, Cell Phones, WiFi, Microwave, Visible Light — all harmless at low intensity. | - AM Radio, FM Radio, Shortwave Radio, TV, Cell Phones, WiFi, Microwave, Visible Light — all harmless at low intensity. | ||
- Near Ultraviolet (UV) — beneficial at low doses (Vitamin D) | - Near Ultraviolet (UV) — ''beneficial'' at low doses (Near UV from the sun produces Vitamin D in the skin.) | ||
- Far UV, X-rays, Gamma-rays — damaging wherever absorbed. (Note that a ''sunburn'' is a ''second-degree radiation burn'' to the skin.) | - Far UV, X-rays, Gamma-rays — damaging wherever absorbed. (Note that a ''sunburn'' is a ''second-degree radiation burn'' to the skin. If it penetrated deeper it could be fatal.) | ||
* '''Fast Massive Particles''': | * '''Fast Massive Particles''': | ||
Line 54: | Line 53: | ||
=What is Ionizing Radiation?= | =What is Ionizing Radiation?= | ||
Radiation is ''ionizing'' if its quantum energy is enough to ionize a neutral atom or molecule, typically 2 eV. For electromagnetic radiation, this means the wavelength must be shorter than half a micron (blue light). So ultraviolet, X-rays and gamma rays can all be ionizing. Cell phones and microwave ovens cannot. Note that ''quantum energy'' (<math>\varepsilon = \hbar \omega </math>) is not the same as the ''intensity'' of the radiation. A microwave produces a huge quantity of very low-energy photons. It will heat your food, but thermal energy is not ionizing until you get up to a few thousand degrees C (when the flame emits light). The sun is 5000 degrees, so if you went there, you would be totally ionized. | Radiation is ''ionizing'' if its quantum energy is enough to ionize a neutral atom or molecule, typically 2 eV. For electromagnetic radiation, this means the wavelength must be shorter than half a micron (blue light). So ultraviolet, X-rays and gamma rays can all be ionizing. Cell phones and microwave ovens cannot. Note that ''quantum energy'' (<math>\varepsilon = \hbar \omega </math>) is not the same as the ''intensity'' of the radiation. A microwave produces a huge quantity of very low-energy photons. It will heat your food, but thermal energy is not ionizing until you get up to a few thousand degrees C (when the flame emits light). The sun is 5000 degrees, so if you went there, you would be totally ionized.<ref>[https://www.omnicalculator.com/physics/photon-energy See Photon Energy]</ref> | ||
Charged particle radiation (alpha, beta) is ionizing, because the particles typically have energies much larger than 2 eV. | Charged particle radiation (alpha, beta) is ionizing, because the particles typically have energies much larger than 2 eV. | ||
Line 64: | Line 63: | ||
=Ionizing Radiation <math>\to</math> DNA Strand Breaks= | =Ionizing Radiation <math>\to</math> DNA Strand Breaks= | ||
The radiation itself rarely damages anything important directly. Instead, it liberates electrons from neutral molecules, leaving behind free radicals that are highly reactive ''chemically''. These radicals can attack DNA molecules and cause "strand breaks" in the double helix that encodes our genetic information. Low doses usually only break one of the strands of a given double helix, but high doses can occasionally break both strands of the same DNA molecule. Our cells contain healing mechanisms to correct such breaks. | |||
* '''Double strand breaks''' are | * '''Single strand breaks''' (SSB) usually heal in milliseconds. According to the NIH, normal oxygen metabolism generates about 10,000 SSB daily in any single mammalian cell. | ||
* '''Double strand breaks''' (DSB) are rarer -- only about a dozen a day per cell due to normal metabolism, plus an extra 1 per cell per ''year'' under 1 mSv/year radiation exposure. DSB take longer to heal, and are occasionally permanent, causing… | |||
- Cell Reproductive Death (most common): | - Cell Reproductive Death (most common): | ||
Cells usually survive for natural lifetimes — a few days for hair follicles, skin and mucous membrane cells; "forever" for neurons and some muscle cells. | Cells usually survive for their natural lifetimes — a few days for hair follicles, skin and mucous membrane cells; "forever" for neurons and some muscle cells. | ||
- Genetic Mutation (most subtle): | - Genetic Mutation (most subtle): | ||
Damaged gamete cells → mutations (usually fatal to foetus; almost always detrimental to | Damaged gamete cells → mutations (usually fatal to foetus; almost always detrimental to the individual offspring) | ||
- Cancer (most unpleasant): Runaway replicative zeal of a misguided cell… | - Cancer (most unpleasant): Runaway replicative zeal of a misguided cell… | ||
Line 90: | Line 91: | ||
=How Bad is How Much of What?= | =How Bad is How Much of What?= | ||
Before we can begin to discuss the effects of radiation, we must have a consistent and reasonably well-defined way of ''measuring the amount'' of radiation delivered. This means we have to define some '''''units''''': | |||
'''Units''': | '''Units''': | ||
• 1 ''rad'' ≡ 100 erg/g (energy deposited per unit mass) | • 1 '''rad''' ≡ 100 erg/g (energy deposited per unit mass) | ||
• 1 '' | • 1 Gray ('''Gy''') ≡ 100 rad ≡ 1 J/kg. [SI units] | ||
Relative Biological Effectiveness ('''RBE''') | Relative Biological Effectiveness ('''RBE'''): | ||
• X-rays, γ-rays & β-rays (fast electrons): RBE = 1 (by definition) | • X-rays, γ-rays & β-rays (fast electrons): RBE = 1 (by definition) | ||
Line 106: | Line 110: | ||
• Fast heavy ions: RBE = 20. | • Fast heavy ions: RBE = 20. | ||
''' | REM ('''R''', ''R''oentgen ''E''quivalent to ''M''an): | ||
• | • R ≡ RBE × rad. | ||
• (1 mR ≡ milliREM] ≡ | • (1 mR ≡ milliREM] ≡ 10<sup>−3</sup> R.) | ||
• Sievert (Sv) [''S''tandard ''I''nternational unit]: 1 Sv ≡ 100 REM | • Sievert ('''Sv''') [''S''tandard ''I''nternational unit]: 1 Sv ≡ 100 REM | ||
Note that we have already introduced a rather empirical "fudge factor" (''RBE''). We have also left out what may be the most important qualifier: the '''''time''''' over which the above ''net doses'' are delivered. It is quite common for people to discuss accumulated doses as if that were all that matters. Some even refer to radiation ''intensity'' levels in mR or µSv as if it didn't matter whether you got your dose in a year or every minute! This is simply ''wrong'' -- the time scale ("per second" or "per hour" or "per day" or "per year") is an essential part of the specification of intensity. | |||
=EFFECTS= | =EFFECTS= | ||
• '''Instant Death''': ∼ 5000 R (50 Sieverts [Sv]) “whole-body” wipes out central nervous system (CNS) | All these effects assume the net dose is delivered within a few hours (usually referred to as "''all at once''"). Unfortunately, many people fail to understand this. If the time scale didn't matter, over our lifetimes we would all exceed the annual legal limit for radiation workers (see below), just from cosmic rays and natural background. | ||
• '''Instant Death''': ∼ 5000 R (50 Sieverts [Sv]) “whole-body” wipes out central nervous system (CNS) ''when delivered all at once'' (''i.e.'' within a few hours). | |||
• '''Overnight Death''': ∼ 900 R (9 Sv) whole-body usually accomplishes the same thing in about a day. | • '''Overnight Death''': ∼ 900 R (9 Sv) whole-body usually accomplishes the same thing in about a day. | ||
• '''Ugly Death''': ∼ 500 R (5 Sv) → severe radiation sickness (nausea, hair loss, skin lesions, etc.) as short-lived cells fail to provide new generations to replace their normal mortality. Complications (infection) | • '''Ugly Death''': ∼ 500 R (5 Sv) → severe radiation sickness (nausea, hair loss, skin lesions, ''etc''.) as short-lived cells fail to provide new generations to replace their normal mortality. Complications (infection) often kill. Some recover completely but may develop leukemia years later; offspring (if any) might have genetic mutations. | ||
• '''Sub-Acute Exposures''': ∼ 100 R (1 Sv) whole-body delivered in less than 1 week → no immediate symptoms, but | • '''Sub-Acute Exposures''': ∼ 100 R (1 Sv) whole-body delivered in less than 1 week → no immediate symptoms, but a possibility of leukemia in later years and/or (rarely) genetic mutations in offspring. | ||
• '''Marginal Exposures''': average exposure in USA from natural & medical sources ∼ 620 mR (6.2 mSv) per year. (The current federal occupational limit of exposure per year for an adult (the limit for a worker using radiation) in the USA is "as low as reasonably achievable; however, not to exceed 5,000 mR [50 mSv]" above the 300+ mR of natural sources of radiation and any medical radiation.) | • '''Marginal Exposures''': average exposure in USA from natural & medical sources ∼ 620 mR (6.2 mSv) per year. (The current federal occupational limit of exposure per year for an adult (the limit for a worker using radiation) in the USA is "as low as reasonably achievable; however, not to exceed 5,000 mR [50 mSv]" above the 300+ mR of natural sources of radiation and any medical radiation.) | ||
Different body parts have dramatically different resistance to radiation. ''Hands'' can withstand radiation doses that would ''kill'' if delivered to the whole body. The ''gonads'' and the ''lens of the eye'' are considered to be the most vulnerable. | |||
Note that for long-lived cells like ''neurons'' and ''muscle'' cells, cumulative doses over times as long as a year have limited meaning in light of the typical time scale of DNA strand-break healing. (See Ref. 2.) However, short-lived cells like ''skin'' cells replicate fast enough that even a partially damaged cell can sometimes propagate itself into a local "precancerous" population that is then vulnerable to subsequent damage leading to malignant cancer. For this reason the most common type of dangerous radiation-induced cancer is the skin cancer '''melanoma''', which is strongly associated with sun exposure. In the USA, the rate of melanoma diagnoses is 78,000 per year or 21.8 per 100,000. The mortality rate is 11.5%. | |||
There is some evidence (''e.g.'' from [https://en.wikipedia.org/wiki/Ramsar,_Mazandaran#Radioactivity Ramsar, Iran]) for '''[https://en.wikipedia.org/wiki/Radiation_hormesis radiation hormesis]''' (marginal exposures may actually promote better health). | There is some evidence (''e.g.'' from [https://en.wikipedia.org/wiki/Ramsar,_Mazandaran#Radioactivity Ramsar, Iran]) for '''[https://en.wikipedia.org/wiki/Radiation_hormesis radiation hormesis]''' (marginal exposures may actually promote ''better'' health). | ||
=Sources of Ionizing Radiation= | =Sources of Ionizing Radiation= | ||
Line 136: | Line 144: | ||
the ''average '''annual''''' doses to USA population have the following sources and magnitudes: | the ''average '''annual''''' doses to USA population have the following sources and magnitudes: | ||
[https://www.epa.gov/sites/default/files/2017-04/donut-pie-chart.png See EPA pie chart.] | <center>[https://www.epa.gov/sites/default/files/2017-04/donut-pie-chart.png See EPA pie chart.]</center> | ||
Note that ''cosmic rays'' make a significant contribution to these accumulated doses. | Note that ''cosmic rays'' make a significant contribution to these accumulated doses. | ||
Line 148: | Line 156: | ||
given that the only damage that matters (DNA strand breaks) usually '''''heals''''' | given that the only damage that matters (DNA strand breaks) usually '''''heals''''' | ||
on a time scale of ''less than'' days, the above numbers are almost meaningless in terms of health risk -- | on a time scale of ''less than'' days, the above numbers are almost meaningless in terms of health risk -- | ||
except for sun damage to the skin, which '''''is''''' accumulative. | except for sun damage to the skin, which '''''is''''' sort of accumulative. | ||
More useful are the "''all at once''" exposures we are apt to encounter: | More useful are the "''all at once''" exposures we are apt to encounter: | ||
Line 181: | Line 189: | ||
releasing the chemically inert noble gas ''radon'', itself a radioactive element | releasing the chemically inert noble gas ''radon'', itself a radioactive element | ||
which emits a low energy α (difficult to detect). | which emits a low energy α (difficult to detect). | ||
Radon probably killed Madame Curie. | Radon probably killed Madame Curie, although it has since been used | ||
widely as a curative treatment for various ailments.<ref name=Radon>One Century of Radon Therapy [http://dx.doi.org/10.1504/IJLR.2004.005434]</ref> | |||
Radon exposure is widespread and dangerous because it accumulates | Radon exposure is widespread and dangerous because it accumulates | ||
in the air of any building made of rock, brick or concrete | in the air of any building made of rock, brick or concrete | ||
(especially those with closed air circulation) | (especially those with closed air circulation) | ||
and thence in the lungs of the people breathing that air, | and thence in the lungs of the people breathing that air, | ||
who become radioactive (easy to detect). | who become radioactive (and thus easy to detect). | ||
Different regions have a huge range of radium content, so a stone | Different regions have a huge range of radium content, so a stone | ||
house may be perfectly safe in one place and hazardous in another. | house may be perfectly safe in one place and hazardous in another. | ||
Line 203: | Line 212: | ||
lungs or other tissues exposes (only) nearby tissue to a huge dose. | lungs or other tissues exposes (only) nearby tissue to a huge dose. | ||
Putin's signature poison <ref name=Putin/> '''polonium''': <SUP>210</SUP>Po is made in reactors by adding | |||
neutrons to bismuth. It is an even deadlier poison. | neutrons to bismuth. It is an even deadlier poison. | ||
Line 244: | Line 253: | ||
=Notes and References= | =Notes and References= | ||
{{Reflist|refs= | |||
<ref name=Putin>Russia Fatally Poisoned A Prominent Defector In London, A Court Concludes [https://www.npr.org/2021/09/21/1039224996/russia-alexander-litvinenko-european-court-human-rights-putin ''NPR 2021'']</ref> | |||
}}[[Category:Suggestion Bot Tag]] |
Latest revision as of 16:00, 9 October 2024

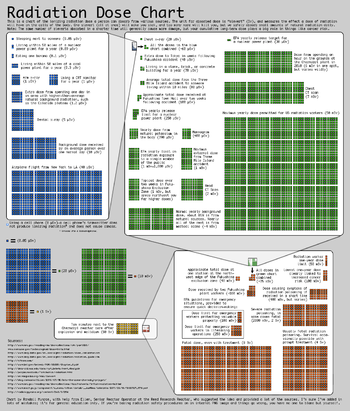
Radiation hazards are not as easy to understand as anyone would like. A primary obstacle to gaining public acceptance for reconsidering improved nuclear power is a generalized fear of radiation. People have difficulty distinguishing between a little and a lot, so that this has often been oversimplified to, "The only acceptable amount of radiation is zero!", a goal that cannot be met because we are constantly subjected to natural radiation over which we have little control, and because all living beings are themselves inherently somewhat radioactive. A sensible understanding of radiation dangers needs to be based on the type of radiation, its intensity, and the time over which the exposure is delivered. This article is an attempt to describe what sorts of damage which kinds of radiation can do, how much exposure over how long a time is required to damage human health, and how quickly the damage caused by radiation can heal.[1]
Figure 1 compares a range of radiation exposures, from the dose received from sleeping next to another human for a night (0.05 Sv) to the usually fatal dose of 4 Sv, a hundred million times greater. Warning: such comparisons can be misleading unless one understands that some are "delivered all at once" and others are "accumulative" over times up to a year. There is great controversy over the latter, since DNA usually "heals" strand-breaks due to free radicals produced by ionizing radiation (see Fig. 2) within hours or days,[2] suggesting that whole-body doses may not "accumulate" over longer periods.
Mortality
Paraphrased from memory: Front page of special HEALTH edition of the LA Free Press (around 1970):
"No matter how much money you have, how good your health care provider or how healthy your diet and life style, you, personally, are going to die."
(I don't really want to believe this any more than you do, but I have to confess that the evidence for it is persuasive.)
Either way, it makes an excellent starting point for any discussion of hazards. We cannot (for now) avoid dying, but we can exercise some influence over when we will die and from what causes. We also (for now) get to choose how much of our enjoyment of life and liberty we sacrifice to this effort.
What is "Radiation"?
There are many kinds of "rays" and waves that act like tiny particles, carrying a large amount of kinetic energy for their size and traveling at or near the speed of light, that are termed "radiation" in this context. Whether they constitute a "hazard" depends on their charge, mass and energy. They fall into the following general groups:
- Electromagnetic Waves:
- AM Radio, FM Radio, Shortwave Radio, TV, Cell Phones, WiFi, Microwave, Visible Light — all harmless at low intensity.
- Near Ultraviolet (UV) — beneficial at low doses (Near UV from the sun produces Vitamin D in the skin.)
- Far UV, X-rays, Gamma-rays — damaging wherever absorbed. (Note that a sunburn is a second-degree radiation burn to the skin. If it penetrated deeper it could be fatal.)
- Fast Massive Particles:
- Electrons (-rays)
- Protons & Neutrons
- -rays (4He nuclei)
- Heavy Ion Beams
- What does the Damage?
- Enough intensity of anything will "cook" you.
- Highly reactive free radicals produced by (among other things) Ionizing Radiation.
What is Ionizing Radiation?
Radiation is ionizing if its quantum energy is enough to ionize a neutral atom or molecule, typically 2 eV. For electromagnetic radiation, this means the wavelength must be shorter than half a micron (blue light). So ultraviolet, X-rays and gamma rays can all be ionizing. Cell phones and microwave ovens cannot. Note that quantum energy () is not the same as the intensity of the radiation. A microwave produces a huge quantity of very low-energy photons. It will heat your food, but thermal energy is not ionizing until you get up to a few thousand degrees C (when the flame emits light). The sun is 5000 degrees, so if you went there, you would be totally ionized.[3]
Charged particle radiation (alpha, beta) is ionizing, because the particles typically have energies much larger than 2 eV.
Neutrons cause ionization indirectly. Slow (thermal) neutrons can be absorbed by a stable nucleus, activating it to a radioisotope that later decays by emitting an ionizing charged particle or a gamma ray. Fast (high energy) neutrons cause "secondary ionization" by colliding elastically with charged nuclei (especially hydrogen) which recoil with enough energy to ionize other atoms. Fast neutrons usually do more damage than slow neutrons.
- What sort of damage?
Ionizing Radiation DNA Strand Breaks
The radiation itself rarely damages anything important directly. Instead, it liberates electrons from neutral molecules, leaving behind free radicals that are highly reactive chemically. These radicals can attack DNA molecules and cause "strand breaks" in the double helix that encodes our genetic information. Low doses usually only break one of the strands of a given double helix, but high doses can occasionally break both strands of the same DNA molecule. Our cells contain healing mechanisms to correct such breaks.
- Single strand breaks (SSB) usually heal in milliseconds. According to the NIH, normal oxygen metabolism generates about 10,000 SSB daily in any single mammalian cell.
- Double strand breaks (DSB) are rarer -- only about a dozen a day per cell due to normal metabolism, plus an extra 1 per cell per year under 1 mSv/year radiation exposure. DSB take longer to heal, and are occasionally permanent, causing…
- Cell Reproductive Death (most common): Cells usually survive for their natural lifetimes — a few days for hair follicles, skin and mucous membrane cells; "forever" for neurons and some muscle cells.
- Genetic Mutation (most subtle): Damaged gamete cells → mutations (usually fatal to foetus; almost always detrimental to the individual offspring)
- Cancer (most unpleasant): Runaway replicative zeal of a misguided cell…
Cancer Therapy
Most cancer radiation therapies involve radiation insults that inflict DNA damage similar to that caused by "bad" radiation.
Strategy: to kill every single cancer cell (along with up to 90% of nearby normal cells).
Dose may be more than 10,000 times maximum legal limit for "accidental" radiation exposure… but are normally not "whole body".
Why Worry, and When? (Informed Consent vs. Public Policy)
Cost/Benefit Analyses: Every public policy decision creates risks. Is this likely to do any good? How much good? Is it likely to do any harm? How much harm? What are the relative probabilities of good and harm? How many people are likely to suffer from the harm? How many people are likely to benefit from the good? And of course the two questions most important to politicians: "Which people?" and "When?"
Any sensible policy regarding radiation hazards, whether public or personal, must take into account that each of us is going to die, that our lifespan is frustratingly short no matter what we do, and that our chances of dying of cancer (radiation-induced or otherwise) are already rather high. (Men have just over a 20% risk of dying from cancer, while women's risk is just over 18%.)
How Bad is How Much of What?
Before we can begin to discuss the effects of radiation, we must have a consistent and reasonably well-defined way of measuring the amount of radiation delivered. This means we have to define some units:
Units:
• 1 rad ≡ 100 erg/g (energy deposited per unit mass)
• 1 Gray (Gy) ≡ 100 rad ≡ 1 J/kg. [SI units]
Relative Biological Effectiveness (RBE):
• X-rays, γ-rays & β-rays (fast electrons): RBE = 1 (by definition)
• Slow neutrons: average RBE ≈ 3. (Variable!)
• Fast neutrons, protons & α-rays: RBE = 10.
• Fast heavy ions: RBE = 20.
REM (R, Roentgen Equivalent to Man):
• R ≡ RBE × rad.
• (1 mR ≡ milliREM] ≡ 10−3 R.)
• Sievert (Sv) [Standard International unit]: 1 Sv ≡ 100 REM
Note that we have already introduced a rather empirical "fudge factor" (RBE). We have also left out what may be the most important qualifier: the time over which the above net doses are delivered. It is quite common for people to discuss accumulated doses as if that were all that matters. Some even refer to radiation intensity levels in mR or µSv as if it didn't matter whether you got your dose in a year or every minute! This is simply wrong -- the time scale ("per second" or "per hour" or "per day" or "per year") is an essential part of the specification of intensity.
EFFECTS
All these effects assume the net dose is delivered within a few hours (usually referred to as "all at once"). Unfortunately, many people fail to understand this. If the time scale didn't matter, over our lifetimes we would all exceed the annual legal limit for radiation workers (see below), just from cosmic rays and natural background.
• Instant Death: ∼ 5000 R (50 Sieverts [Sv]) “whole-body” wipes out central nervous system (CNS) when delivered all at once (i.e. within a few hours).
• Overnight Death: ∼ 900 R (9 Sv) whole-body usually accomplishes the same thing in about a day.
• Ugly Death: ∼ 500 R (5 Sv) → severe radiation sickness (nausea, hair loss, skin lesions, etc.) as short-lived cells fail to provide new generations to replace their normal mortality. Complications (infection) often kill. Some recover completely but may develop leukemia years later; offspring (if any) might have genetic mutations.
• Sub-Acute Exposures: ∼ 100 R (1 Sv) whole-body delivered in less than 1 week → no immediate symptoms, but a possibility of leukemia in later years and/or (rarely) genetic mutations in offspring.
• Marginal Exposures: average exposure in USA from natural & medical sources ∼ 620 mR (6.2 mSv) per year. (The current federal occupational limit of exposure per year for an adult (the limit for a worker using radiation) in the USA is "as low as reasonably achievable; however, not to exceed 5,000 mR [50 mSv]" above the 300+ mR of natural sources of radiation and any medical radiation.)
Different body parts have dramatically different resistance to radiation. Hands can withstand radiation doses that would kill if delivered to the whole body. The gonads and the lens of the eye are considered to be the most vulnerable.
Note that for long-lived cells like neurons and muscle cells, cumulative doses over times as long as a year have limited meaning in light of the typical time scale of DNA strand-break healing. (See Ref. 2.) However, short-lived cells like skin cells replicate fast enough that even a partially damaged cell can sometimes propagate itself into a local "precancerous" population that is then vulnerable to subsequent damage leading to malignant cancer. For this reason the most common type of dangerous radiation-induced cancer is the skin cancer melanoma, which is strongly associated with sun exposure. In the USA, the rate of melanoma diagnoses is 78,000 per year or 21.8 per 100,000. The mortality rate is 11.5%.
There is some evidence (e.g. from Ramsar, Iran) for radiation hormesis (marginal exposures may actually promote better health).
Sources of Ionizing Radiation
According to the 2021 EPA survey, the average annual doses to USA population have the following sources and magnitudes:
Note that cosmic rays make a significant contribution to these accumulated doses. Fortunately our atmosphere makes an excellent shield; but since it is most dense near sea level, people living at high altitude (like Denver) receive a higher annual dose, and flying at 40,000 feet delivers about 0.7 mR per hour.
NOTE: Since rates of exposure vary constantly, the temptation to average over a year is understandable; however, for the majority of cell types, given that the only damage that matters (DNA strand breaks) usually heals on a time scale of less than days, the above numbers are almost meaningless in terms of health risk -- except for sun damage to the skin, which is sort of accumulative.
More useful are the "all at once" exposures we are apt to encounter:
• Medical X-rays:
- Whole Body CT scan: 1,000 mR (10 mSv) per exposure
- Upper GI X-ray: 600 mR (6 mSv) per exposure
- Head CT scan: 200 mR (2 mSv) per exposure
- Mammogram: 42 mR (0.42 mSv) per exposure
- Chest X-ray: 10 mR (0.1 mSv) per exposure
Natural Terrestrial Radionuclides
Gamma (γ) radiation is fairly uniform in the USA, ranging from 30 mR/y in Texas to 115 mR/y in South Dakota.
The Really Bad Stuff: Ingested Radionuclides
Many radionuclides (radioactive isotopes) emit fast α particles (4He nuclei). The range of most α "rays" is only on the order of cm in air and mm in tissue. This is good if they are at arm's length; but it's bad if you swallow them or breathe them! A wide variety of radioactive elements have assorted chemical properties, each with a specific hazard.
• Radon: All rock contains some radium which slowly decays, releasing the chemically inert noble gas radon, itself a radioactive element which emits a low energy α (difficult to detect). Radon probably killed Madame Curie, although it has since been used widely as a curative treatment for various ailments.[4] Radon exposure is widespread and dangerous because it accumulates in the air of any building made of rock, brick or concrete (especially those with closed air circulation) and thence in the lungs of the people breathing that air, who become radioactive (and thus easy to detect). Different regions have a huge range of radium content, so a stone house may be perfectly safe in one place and hazardous in another.
• Potassium & Carbon: Radioisotopes of K and C are continually created in the atmosphere by cosmic ray bombardment and build up to a constant level in all living tissues, only to decay away in a few thousand years after death. You are radioactive! Potassium-Argon and 14C dating provides handy means of estimating how long ago biological matter was alive.
• Man-Made Radionuclides: Formerly most famous: plutonium, 239Pu, of which fission bombs are made. A deadly chemical poison as well as a nasty radioisotope, a miniscule grain of 239Pu caught in your lungs or other tissues exposes (only) nearby tissue to a huge dose.
Putin's signature poison [5] polonium: 210Po is made in reactors by adding neutrons to bismuth. It is an even deadlier poison.
The food chain concentrates "harmless" levels to dangerous ones; fission waste products include many radionuclides with chemical properties not seen in naturally occurring isotopes. Otherwise it might be marginally sensible to dispose of radioactive waste by diluting it in the oceans. Although radioisotopes from the Fukushima meltdown were easily detected on the Pacific coast of North America, the levels were utterly harmless.
It's important to understand the difference between "detectable" and "dangerous".
In the 1960s we could detect parts per million (ppm) of Selenium (Se), a heavy metal which is poisonous at that concentration. The US Congress then passed a law making it illegal for any foodstuffs to contain a detectable amount of Se. A few years later we were able to detect parts per billion (ppb). That’s when we discovered that Se is an essential mineral. This illustrates the foolishness of any policy of "zero tolerance". The current policy regarding radiation exposure from nuclear reactors is ALARA ("As Low As Reasonably Achievable"), which is routinely interpreted as "zero tolerance" even though there is no evidence for significant deleterious effects of marginal exposures, and even some evidence for beneficial effects ("radiation hormesis").
Protection Against Radiation
The best shielding is provided by Gauss' Law: intensity ∝ 1/r2. Localized sources are labelled with their activity at a given distance, for instance "10 mR/h at 1 m", which becomes 0.1 mR/h at 10 m. Just walk away! By the same token, never touch a radioactive source! (1/r2 → ∞ as r → 0).
Lead aprons are effective only for X-rays, γ-rays and low energy β-rays. Thick concrete shielding is needed for neutrons and high-energy charged particles.
For ingested radionuclides, chelation can sometimes help. In the case of tritium (3H), one should drink lots of beer!
Notes and References
- ↑ Lecture on Radiation Hazards Jess H. Brewer
- ↑ "Effects of Ionizing Radiation on Biological Molecules—Mechanisms of Damage and Emerging Methods of Detection", Julie A. Reisz, Nidhi Bansal, Jiang Qian, Weiling Zhao, and Cristina M. Furdui doi: 10.1089/ars.2013.5489
- ↑ See Photon Energy
- ↑ One Century of Radon Therapy [1]
- ↑ Russia Fatally Poisoned A Prominent Defector In London, A Court Concludes NPR 2021