Halobacterium NRC-1

For the course duration, the article is closed to outside editing. Of course you can always leave comments on the discussion page. The anticipated date of course completion is May 21, 2009. One month after that date at the latest, this notice shall be removed. Besides, many other Citizendium articles welcome your collaboration! |
Halobacterium sp. NRC-1 | ||||||||||||||
---|---|---|---|---|---|---|---|---|---|---|---|---|---|---|
![]() | ||||||||||||||
Scientific classification | ||||||||||||||
| ||||||||||||||
Binomial name | ||||||||||||||
Halobacterium sp. NRC-1 |
Description and Significance
Halobacterium sp. NRC-1 is a non-pathogenic, halophilic archaea which thrives all over the world in high salt environments, including salt production facilities, brine inclusions in salt crystals, natural lakes and ponds, and salt marshes. Prior to 1990 H. NRC-1 was classified as an archeabacterium under the prokaryote kingdom in the two-empire system which consisted of eukaryotes and prokaryotes. Since 1990 the prokaryotes were split into bacteria and archaea due to their different evolutionary paths and biochemical differences.[1] Like all archaea H. NRC-1 has no nucleus or organelles within the cell, and like other archaea, have evolved many metabolic pathways to allow it to survive in extreme environments.[2] Halobacterium sp. NRC-1 is motile using both flagella and gas vesicles, and respond to their environment by moving towards chemicals using a process called chemotaxis and toward or away from light using phototaxis using its sensory rhodopsins. They reproduce via binary fission and grow best in a 42 degree Celsius aerobic high salt environment.
Halobacterium sp. NRC-1 is very easy to culture in the lab and its genome has been completely mapped. Whole-genome DNA microarrays are available to investigate gene expression. This makes it an excellent model microorganism for research into the basic cellular process and gene expression as well as for teaching.
Genome structure
The genome of Halobacterium sp. NRC-1 was published in 2000. Since that time scientists have done extensive research to gain insights into both its extremophilic abilities as well as its biological processes. In order to survive in its environment, H. NRC-1 has developed amazing capabilities to repair its own genome, one being a precise base excision repair system. Homologous recombination plays an important role in DNA repair as well. Its ability to repair its chromosomes after extensive damage is only exceeded by the extremely radiation resistant Deinococcus radioduransbacterium. In fact, H. NRC-1 was found to display a significant number of unique homologs with this bacterium, which suggests that they might have been acquired through lateral gene transfer.[3] This microorganism also displays other types of defense mechanisms. In combination with its saline environment which provides some protection from UV radiation, the proteins that are produced by H. NRC-1 were found to be highly acidic. This low pH helps the proteins resist the denaturing effects of the high concentration of salts that surrounds it.[4][5][3]
Halobacterium sp. NRC-1 contains the smallest genome to date among the halophiles.[6]
It is 2,571,010 bp in size, and is composed of a large GC-rich chromosome (2,014,239 bp, 68 % G+C), and two smaller extrachromosomal replicons, pNRC100 (191,346 bp) and pNRC200 (365,425 bp), with 58–59 % G+C composition. The large chromosome contains 2,111 genes, pNRC200 contains 374, and pNRC100 contains 197. Of the 2,682 genes in the genome, only 1,658 coded for proteins that had significant matches to the genome database. A significantly smaller fraction of the genes on pNRC200 and pNRC100 matched to genes of known function in the databases. The genes on the large chromosome had a 45% match rate, while pNRC200 had a 32% match rate and pNRC100 had only 26% of it's genome match up to genes of known function. 52 RNA genes have also been identified. Interestingly, about 40 genes that are located on the small replicons, pNRC100 and pNRC200, code for functions likely to be essential or important for cell livelihood (e.g. thioredoxin and thioredoxin reductase, a cytochrome oxidase, a DNA polymerase, multiple TATA-binding proteins (TBP) and transcription factor B (TFB) transcription factors, and the only arginyl-tRNA synthetase in the genome. In fact, these two replicons share 145,428 base pairs of identical DNA.[3]
After the publication of Halobacterium sp. NRC-1 complete genome, the mapping of another closely related Halobacterium, Halobacterium sp. Salinarum, was accomplished as well. Interestingly, genomic comparisons between these two halophiles showed that the large chromosomes were virtually identical to one another while the smaller replicons carried different genes. This information casted new light on how the species differ from each other and the role of replicons in these organisms. While much of the published literature on Halobacterium sp. NRC-1 refers to the smaller genetic elements as replicons or megaplasmids, their characteristics don't really fit the definition of these terms. Replicons are considered to be exact copies of specific sequences of an original DNA or RNA genome, or even a whole copy of the original genome. Plasmids are referred to as being small extra chromosomal DNA elements that carry relatively few genes that code for genetic information that is not essential to an organism's biological processes. Considering that the genetic information carried on these replicons not only code for information that is essential to the organism's survival, but also contain nucleotide sequences that are not identical to that of it's larger chromosome, scientists are beginning to refer to them as "minichromosomes" rather than megaplasmids or replicons.
Cell structure and metabolism
Halobacterium sp.NRC-1 is a rod shaped aerobic/facultative anaerobic chemoorganotroph which uses organic molecules as its source of energy, carbon and electrons. Research has shown that it is unable to metabolize sugars, but instead rely on amino acids that are eventually catabolized by the citric acid cycle during aerobic respiration. It survives on the remains of less halophilic organisms that have lysed due to the overwhelming amounts of salt in the environment. Outside of their natural environment, cells are cultured best in a complex medium. A minimal medium described for Halobacterium includes all but 5 of the 20 amino acids for growth.[3]
This microorganism has been extensively studied and shown to contain some of the usual features found in halophilic archaea, for example, an S-layer glycoprotein, ether-linked lipids, and purple membrane.[7] This purple membrane consists of the light-driven ion transporters bacteriorhodopsin and halorhodopsin, and the phototaxis receptors, sensory rhodopsins I and II.[3] In order to survive in low oxygen environments, Halobacterium sp. NRC-1 switches to anaerobic respiration and increases its production of Bacteriorhodopsin, which is a unique protein that can use light as an energy source, much like chlorophyll can in cyanobacteria and phototrophic eukaryotes. When the retinal in in Bacteriorhodopsin absorbs light, it results in a series of conformational changes that translocates a proton through the cell's membrane into the periplasmic space. This light driven proton pumping generates an electrochemical proton gradient which is then used to power the synthesis of ATP. This phototrophic capability is particularly useful to Halobacterium sp. NRC-1 as oxygen is not very soluble in concentrated salt solutions. In addition to its phototrophic respiration capabilities,it is also capable of anaerobic respiration using dimethyl sulfoxide (DMSO) and trimethylamine-N-oxide (TMAO) as terminal electron acceptors. Arginine can also be used in the process of fermentation.[8]
Halobacterium sp. NRC-1 is also classified as an obligate halophilic microorganism which has adapted to be able to grow in conditions of extremely high salinity, up to 10 times that of seawater.[3] In order to survive under these conditions it maintains a very high concentration of salts internally in the form of KCl to enable it to remain isotonic to it's preferred environment.[9] Halorhodopsin plays a very important role in helping to maintain the osmotic balance within the cell. This membrane protein acts as a light driven pump by transporting chloride and potassium ions into the cell. Halorhodopsin saves the organism a large amount of metabolic energy by using the energy of the yellow light that it captures to fuel the movement of these ions.[10]
Ecology
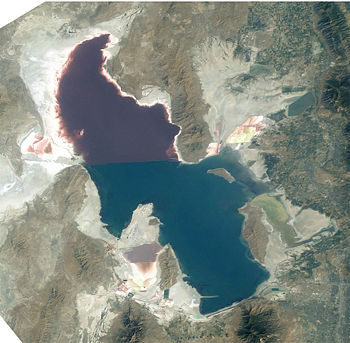
Halobacterium sp. NRC-1 is one of many strains of halobacterium which thrive in extremely high salinity environments such as salt lakes, salt marshes and salt drying ponds. Their optimal temperature for reproduction is 42°C. Often these highly saline bodies of water will be tinted red or purple. It is the red/purple color of the bacteriorhodopsins that give the red color you often see in these highly saline environments. Bacteriorhodopsin consists of a photosensitive protein pigment called retinal. This protein pigment is responsible for NRC-1's colorful properties. The more saline the environment the more purple the color will be because halobacterium increase their production of bacteriorhodopsin in response to drops in oxygen which is less soluble in saline solutions. There are not many other organisms that can survive in these high salt environments, in fact one of its primary sources of food is the amino acids of other organisms which have lysed due to the high salt concentration in this environment. Brine shrimp are one of a few other organisms that can survive the high salt concentration, and they feed almost exclusively on the bacteria in their environment. Interestingly, the flamingo, whose pink color comes exclusively from its diet (it doesn't have the ability to make the carotenoids which give the pink color) feeds on the brine shrimp, so the carotenoids which give the flamingo its pink color actually come from the carotenoids in the halobacterium that the shrimp eat and whatever carotenoids that the shrimp produce naturally.
Application to Biotechnology
Halobacterium NRC-1 can easily be analyzed just by placing it in a hypotonic solution. Due to the higher salt concentration within the microorganism in comparison to it's less salinic environment, water will flow into the cell following its concentration gradient, causing the cell to swell and and undergo lysis. This explosion releases this microorganism's proteins, which then can be used by researchers for genetic analysis. Halobacterium sp. NRC-1 was one of the first Archaea to have its genome fully mapped and published. Since that time several more species have been successfully mapped and a few others partially mapped. This allows scientists to analyze the properties of halobacterium in silico to determine the activity of genes. Many transformation tools have also been developed to create loss-of-function mutants. These mutant strains are made to carry inoperative knockout genes which alters the organism's normal cellular processes. Scientists can then infer the function of the gene by comparing the physical and biochemical characteristics of the original organism with the new mutant's characteristics. [11] The ability of this organism to be easily cultured along with these ingenious genetic research tools make Halobacterium sp. NRC-1 an ideal model for testing the function of genes.
In addition, Halobacterium sp. NRC-1 is an ideal study tool because it is an extremophile, it exists in environments that are very unfriendly toward life. This makes it very useful for studying many biological questions especially those involved with adaptation and survival in extreme environments. In fact, much research is being done to investigate whether or not halobacterium could be potential candidates for extraterrestrial life, such as on Mars or Europa. Recent research has shown that Halobacterium sp. NRC-1 can not only survive at temperatures far below its optimal growth temperature, but continue to reproduce as well.[12]
Halobacterium sp. NRC-1 is also playing a significant role in the advancement of immunological biotechnology. Recent experimental findings show that recombinant gas vesicles from a mutant strain of Halobacterium sp. NRC-1 have the potential to serve as antigen display/delivery systems. DNA segments of an SIV gene were inserted into a specific site on a GvpC gene (gas vesicle protein C) in the Halobacterium. The gene was successfully taking up by the microorganism and recombination had been accomplished. Subsequently, the gas vesicles began to express the new recombinant/pathogenic proteins at their surface. Mice were then immunized with these SIV recombinant gas vesicles. Antibody production was monitored, and an increased level of humoral response was observed. These results show that the gas vesicles of Halobacterium sp. NRC-1 show great potential in their possible roles as immunizing agents.
Current Research
Survival and growth of Halobacterium sp. NRC-1 following incubation at -15°C, freezing or freezedrying, and the protective effect of cations.
The extremophillic properties and biological responses of Halobacteruim NRC-1 are currently being investigated to test the organism's survival potential in the harsh environmental conditions that exist on Mars. The recent discoveries that meterorites from Mars contain a mineral form of sodium chloride as well as the isolation of halophilic archaea from ancient rock salt has led scientists to believe that the existence of Halobacterium sp. NRC-1 in a Martian environment is quite possible. Halobacterium are known to be slightly thermophilic organisms, showing optimum growth at 40 degrees Celcius. In this experiment, cells of Halobacterium sp. NRC-1 were freeze dried and suspended in buffers containing specific cations, including Mg++,Ca++,or K+. Results showed that NRC-1 was still capable of growth at the extremely low temperature of -15 degrees Celcius, and the cations appeared to show protective characteristics. The conditions of the the Martian environment include very low temperatures and low water availabilty. Scientists will continue to research NRC-1's ability to survive in such inhospitable environments such as the one on Mars.[12]
Functional Genomics of Thioredoxins in Halobacterium sp. NRC-1.
This project addresses the functions of an ancient protein family in Archaea that occupy extreme environments. Some of these proteins may play roles similar to those of comparable proteins in other living organisms, and thus may tell us about functions that evolved in the last universal common ancestor of life. Others may have evolved as the Archaea began to occupy specialized and often extreme environments. This project also addresses the emergence of proto-metabolic networks that supplied the precursors for the RNA World.[13]
A systems view of haloarchaeal strategies to withstand stress from transition metals.
Given that transition metals are essential cofactors in central biological processes, misallocation of the wrong metal ion to a metalloprotein can have resounding and often detrimental effects on diverse aspects of cellular physiology. Therefore, in an attempt to characterize unique and shared responses to chemically similar metals, we have reconstructed physiological behaviors of Halobacterium NRC-1, an archaeal halophile, in sublethal levels of Mn(II), Fe(II), Co(II), Ni(II), Cu(II), and Zn(II).[14]
References
- ↑ Woese, C R (1994-03). "There must be a prokaryote somewhere: microbiology's search for itself". Microbiological Reviews 58 (1): 1-9. ISSN 0146-0749. Retrieved on 2009-04-28.
- ↑ Archaea - Wikipedia, the free encyclopedia. Retrieved on 2009-04-26.
- ↑ 3.0 3.1 3.2 3.3 3.4 3.5 Ng, Wailap Victor, et al. (2000-10-24). "Genome sequence of Halobacterium species NRC-1". Proceedings of the National Academy of Sciences of the United States of America 97 (22): 12176-12181. DOI:- 97 VL - 97. Retrieved on 2009-04-18. - 97 Research Blogging.
- ↑ McCready, Shirley; Jochen Muller, Ivan Boubriak, Brian Berquist, Wooi Ng, Shiladitya DasSarma (2005). "UV irradiation induces homologous recombination genes in the model archaeon, Halobacterium sp. NRC-1". Saline Systems 1 (1): 3. DOI:10.1186/1746-1448-1-3. ISSN 1746-1448. Retrieved on 2009-04-18. Research Blogging.
- ↑ Kottemann, Molly; Adrienne Kish, Chika Iloanusi, Sarah Bjork, Jocelyne DiRuggiero (2005-06-01). "Physiological responses of the halophilic archaeon Halobacterium sp. strain NRC1 to desiccation and gamma irradiation". Extremophiles 9 (3): 219-227. DOI:10.1007/s00792-005-0437-4. Retrieved on 2009-04-28. Research Blogging.
- ↑ DasSarma, Shiladitya; Brian Berquist, James Coker, Priya DasSarma, Jochen Muller (2006). "Post-genomics of the model haloarchaeon Halobacterium sp. NRC-1". Saline Systems 2 (1): 3. DOI:10.1186/1746-1448-2-3. ISSN 1746-1448. Retrieved on 2009-04-18. Research Blogging.
- ↑ Kennedy, S P; W V Ng, S L Salzberg, L Hood, S DasSarma (2001-10). "Understanding the adaptation of Halobacterium species NRC-1 to its extreme environment through computational analysis of its genome sequence". Genome Research 11 (10): 1641-50. DOI:10.1101/gr.190201. ISSN 1088-9051. Retrieved on 2009-04-18. Research Blogging.
- ↑ Müller, Jochen A.; Shiladitya DasSarma (2005-03). "Genomic Analysis of Anaerobic Respiration in the Archaeon Halobacterium sp. Strain NRC-1: Dimethyl Sulfoxide and Trimethylamine N-Oxide as Terminal Electron Acceptors". Journal of Bacteriology 187 (5): 1659–1667. DOI:10.1128/JB.187.5.1659-1667.2005. Retrieved on 2009-04-18. Research Blogging.
- ↑ Coker, James; Priya DasSarma, Jeffrey Kumar, Jochen Muller, Shiladitya DasSarma (2007). "Transcriptional profiling of the model Archaeon Halobacterium sp. NRC-1: responses to changes in salinity and temperature". Saline Systems 3 (1): 6. DOI:10.1186/1746-1448-3-6. ISSN 1746-1448. Retrieved on 2009-04-18. Research Blogging.
- ↑ Oesterhelt, D (1998-08). "The structure and mechanism of the family of retinal proteins from halophilic archaea". Current Opinion in Structural Biology 8 (4): 489-500. ISSN 0959-440X. Retrieved on 2009-04-26.
- ↑ Soppa, Jorg (2006-03-01). "From genomes to function: haloarchaea as model organisms". Microbiology 152 (3): 585-590. DOI:10.1099/mic.0.28504-0. Retrieved on 2009-04-18. Research Blogging.
- ↑ 12.0 12.1 Weidler, Gerhard (2004-03-01), Survival and growth of Halobacterium sp. NRC-1 following incubation at -15°C, freezing or freeze-drying, and the protective effect of cations, Third European Workshop on Exo-Astrobiology, vol. 545, at 311-312. Retrieved on 2009-04-18
- ↑ Library of Resources « NASA Astrobiology. Retrieved on 2009-04-18.
- ↑ Kaur, Amardeep; Min Pan, Megan Meislin, Marc T. Facciotti, Raafat El-Gewely, Nitin S. Baliga (2006-07). "A systems view of haloarchaeal strategies to withstand stress from transition metals". Genome Research 16 (7): 841–854. DOI:10.1101/gr.5189606. Retrieved on 2009-04-18. Research Blogging.